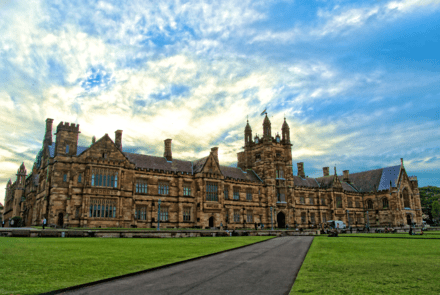
(Wikimedia Commons public domain photo)
We should be thankful that we live, that there exist a planet and a cosmos permitting us to think, to love, and to thank.
Here are three science-related newspaper columns that I published in the Deseret News for past American Thanksgiving holidays:
“The miracle of Thanksgiving pies”
Jeff Lindsay, a vice president of the Interpreter Foundation who happens to hold a doctorate in chemical engineering, has recently posted a quite compatible blog entry, partially science-focused:
“A Most Appropriate Thanksgiving Message for This Day: President Nelson on the Power of Gratitude”
***
I will now continue somewhat in the same vein, with some lines based upon Geraint F. Lewis and Luke A. Barnes, A Fortunate Universe: Life in a Finely Tuned Cosmos (Cambridge: Cambridge University Press, 2016), 47-53,
First, you need to understand that a quark is any one of a number of subatomic particles that carry a fractional electric charge. Although they’ve been postulated as building blocks of the hadron, quarks haven’t actually been directly observed. Still, theoretical predictions based on their existence appear to have been experimentally confirmed. That’s really all the physics that you need to know for what follows:
The up and down quarks are, respectively, 4.5 times heavier than the electron and 9.4 times heavier. These aren’t the most obvious numbers, or the neatest, but they are fundamental to what’s called the Standard Model of particle physics. We can measure them, but we have no explanation for their specific values. Why are they what they are? We don’t know.
Nor does the situation necessarily get any clearer in other regions of the Standard Model. The other four quarks, for instance, are 190 times heavier than the electron, and 2495 times, and 8180 times, and 338,960 times heavier. And the muon and the tau are 206.768,284 and 3477.15 times heavier than the electron, respectively.
Do these particular and rather peculiar values have any special significance? How would the universe change if the relative masses of electrons, quarks, muons, and taus were slightly different? Is life so hardy and robust, so remarkably malleable, that it would simply take a somewhat different form? Will biology arise in virtually any universe?
No.
If we were to increase the mass of the down quark by a factor of about 70, it would very soon decay into what’s called a [Delta]++ particle — sorry, I can’t do Greek characters here — and we would find ourselves in what Lewis and Barnes call a “Delta-Plus-Plus Universe.” Since the extra positive charge would cause electromagnetic repulsion (such as we see when we try to bring the positive sides of two magnets together), such particles would be much less likely to bind to each other. Individual [Delta]++ particles would be able to capture two electrons (because of their negative charges) in order to create an element like helium. But this would be the only element in the universe. “Farewell, periodic table!” say Lewis and Barnes (50). And farewell, life! The universe in which we live permits something like sixty-one million chemical compounds of which we’re currently aware; the “Delta-Plus-Plus Universe” would permit just one element and absolutely no chemical reactions.
But suppose we increase the mass of the up quark by a factor of 130. Protons and neutrons will be replaced by just one kind of very stable particle made up of three down quarks, known as the [Delta]– particle. Within this Delta-minus universe, as Lewis and Barnes label it, with no neutrons to dilute the repulsive force of their powerfully negative charge, there will be just one uniform type of atom. However, there will be, potentially, one possible chemical reaction: If all electrons are replaced with their positively charged alter egos, positrons, two [Delta]-minus particles will be able to form a molecule. But that’s it. No life.
To create a hydrogen-only universe, we increase the mass of the down quark by at least a factor of three. In this hypothetical cosmos, neutrons will vanish. Even those in nuclei. “Once again,” comment Lewis and Barnes, “kiss your chemistry textbook goodbye, as we’d be left with one type of atom and one chemical reaction.” (51) No chemical activity. No biology.
“If you think the hydrogen universe is rather featureless,” write Lewis and Barnes, “let’s instead increase the mass of the up quark by a factor of 6.” (51) If we do this, protons fall apart. All protons decay into neutrons, positrons, and neutrinos. “This is by far the worst universe we’ve so far encountered,” comment Lewis and Barnes, with “no atoms, no chemical reactions. Just endless, featureless space filled with inert, boring neutrons.” (51) In other words, once again, no life.
But there are still other paths by which we might reach this sterile neutron universe. For example, we might decrease the mass of the down quark by just eight percent. In that case, protons in atoms would capture the electrons orbiting them. Atoms would dissolve into featureless neutrons. There would be no chemistry. And thus, no biology.
We’ve had relatively little to say thus far about electrons. But electrons and their antiparticles, positrons, are involved in the decay of neutrons and protons, so electrons, too, can sterilize a universe. For example, if we increase the mass of an electron by a factor of 2.5, we’re back in the completely dead neutron universe again. No life.
On page 52 of their book, Lewis and Barnes offer their Figure 12. (I can’t show it here, but I will attempt to describe it.). It’s about 2.0 inches wide by 3.35 inches tall, with a vertical axis representing the hypothetical masses of up and down quarks and a horizontal axis representing hypothetical electron masses, both measured in MeV or “megaelectronvolts.” Each point within the figure represents a different universe. If the reader throws a dart, the point where the dart hits marks a different hypothetical universe, with different mass ratios between electrons and down and up quarks. The grey regions of the figure show where the complex chemistry required for biological life simply cannot occur, divided between universes dominated only by protons (the hydrogen universe) or only neutrons. On the left side is a small triangular wedge marking the territory of the “acceptable” universes, the hypothetical universes in which potentially life-supporting chemical reactions might possibly occur. It is about 3/4 inch on its base with 1/2 inch sides. In it, there is a black dot that represents the “location” within these parameters of the actual universe that we know. Happily, we’re well within the white wedge. Remark Lewis and Barnes, “This, effectively, is the start of the fine-tuning journey.”
And, indeed, you might be pardoned if you found that relatively small wedge of life-permitting universes — perhaps 0.15 square inch — within a rectangle of seven life-forbidding square inches fairly impressive.
But their final comment on the figure is so significant and so stunning that I’ll simply quote it here:
An important part of this plot to keep a firm eye on is the limits of the axes. We plotted the quark difference from 0 to 7 MeV, and the electron mass from 0 to 4 MeV. This region is the interesting bit of the plot; the rest is boring and grey. If we wanted, instead, to get an impression of the smallness of the white, life-permitting region, we could extend the axes up to the mass of the largest quark we’ve observed: the top quark. To see this, add 4 kilometres of grey paper to the right, top, and bottom of the plot. (If you make this 10,000 acre plot, please send us a photo.)
If we extend to the limits of our experimental data, we’d mark 6.5 million MeV at the ends [of] a plot that could cover most of Tasmania, or all of Switzerland, or half of South Korea, or most of West Virginia, or two Death Stars, depending on which continent/universe you inhabit. (52-53)
#GiveThanks
***
I’ve just read — and thoroughly enjoyed — Fiona and Terryl Givens, All Things New: Rethinking Sin, Salvation, and Everything in Between (Meridian, ID: Faith Matters Publishing, 2020). Here’s one of the many passages that I liked and marked:
As Saints we should have no problem acknowledging the abundant “giftedness” that everywhere colors the Great Plan of Happiness from beginning to end. First, because Christ’s gift precedes rather than follows our need. Creation anticipates our arrival, His Atonement foresees our sorrows and suffering, and His Resurrection inaugurates our own. (154)
The idea that “Creation anticipates our arrival” reminds me very strongly of the famous statement made by the recently departed Anglo-American mathematical physicist Freeman Dyson (1923-2020), of the Institute for Advanced Study at Princeton:
The more I examine the universe and the details of its architecture, the more evidence I find that the universe in some sense must have known we were coming.
#GiveThanks